باربرا مكلينتوك
Charles Darwin
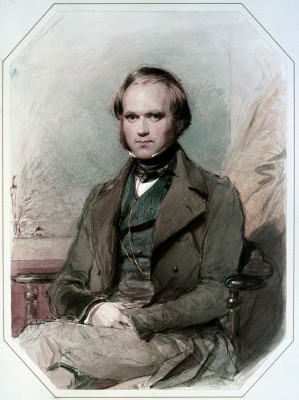
Watercolour of Charles Darwin painted by George Richmond after Darwin’s return from the voyage of HMS Beagle (Image via Wikimedia Commons)
If he had held a passport Charles Robert Darwin (1809-1882) might have been described as a naturalist, but he was one of the greatest of scientists, a titan among biologists. His theory of evolution by natural selection unified biology in its widest sense, providing a coherent explanation for how the diversity of life came to be. Alongside related enquiries it made sense of diverse lines of evidence: how species were related, how the fossil record shed light on vanished worlds, why some organs were vestigial, where our emotions may come from, how oceanic coral reefs formed, and much more besides. This contribution to our understanding of life on Earth has led to Darwin being elevated to the top-most pantheon of great scientists and he remains one of the truly key figures in the history of science. Few scientists have had their career more exhaustively documented.
Darwin had initially intended to follow in his father’s footsteps as a doctor, beginning this career at Edinburgh University. He found, however, the medical work to be repugnant. His fascination for natural history led him to neglect his studies, with his father ultimately sending him to Cambridge to study divinity with the intention of his son becoming an Anglican parson. This, of course, did not happen, but it underlines the often serendipitous nature of science. When Douglas Adams wrote “I may not have gone where I intended to go, but I think I have ended up where I needed to be.” (1988, p.142) he wasn’t referring to Darwin, but it can with some justice be applied to him.
In 1831, one of Darwin’s mentors at Cambridge, the botanist Professor John Stevens Henslow, recommended him as a gentleman companion and naturalist to accompany Captain Robert Fitzroy, who was about to embark on what turned out to be a five-year trip around the world, on HMS Beagle. This experience had a profound impact upon Darwin and formed the basis for many of the observations he used to develop his seminal publication On the Origin of Species.
On the Origin of Species was published in 1859 and sold out immediately. Today it is available in almost every language (including Esperanto), and within Darwin’s lifetime ran to six editions. An insight into its impact, as well as to Darwin’s scientific integrity, is relayed by Desmond and Moore (1991, p.582). They describe how, upon hearing that working men in Lancashire were clubbing together in order to afford a copy of Origin of Species, Darwin insisted that the cost of the book be reduced because he wanted it to be available to everyone. Its fundamental thesis was the ground-breaking idea of evolution by natural selection. As is well-known, the germ of this idea derived from the economist Thomas Malthus, but in Darwin’s hands the concept of natural selection provided a cogent explanation as to how adaptations occurred and, by implication, species arose.
Yet so familiar is the idea of natural selection that its sheer explanatory power has on occasion led to it becoming misunderstood. So it is that natural selection is sometimes thought of as a ‘force’ seeking out to destroy ‘stupid’ individuals. For example, images of an individual carrying out some foolish act (such as diving into an empty swimming pool) are tagged with a heading along the lines of “Natural selection in progress”. One needs to remember that natural selection is not a ‘force’ striving to produce ‘progress’, a balanced ecosystem, or for that matter anything else. Rather, it is the consequence of differential ratio of reproduction, and as such is mechanistic.
Natural selection, therefore, has neither fore-knowledge nor a particular goal in mind. Put simply, natural selection is a process by which biological traits increase or decrease in a population because some individuals have more offspring than others.
It is also important to note that whilst natural selection is a key mechanism of evolution, it is by no means the only one. Thanks largely to the field of work founded by the botanist Gregor Mendel (regarded as the father of genetics) we know that evolution is also driven by genetic mutation, gene duplication, migration, genetic drift, and other factors. Broadly speaking, whilst evolution is universally accepted, the field of neo-Darwinism continues to explore not only natural selection but a range of other mechanisms that lead to the diversity of life we see around us.
A second matter that perhaps needs clarification is the word ‘theory’. This is because anti-evolutionists attempt to undermine evolution on the grounds that it is “only a theory”. This stems from a misunderstanding of what is meant by the scientific idea of a ‘theory’. In everyday life ‘theory’ tends to mean an unsubstantiated opinion or the postulated outcome of an event or hunch. In this way the word ‘theory’ is often used interchangeably with the word ‘guess’. However, in the scientific world, a theory is an overarching explanation for an aspect of the natural world that is supported by hypothesis-driven evidence.
One does not need to subscribe to the idea of paradigm shifts (in the manner of physicist Thomas Kuhn) or that science is dependent on a cultural context (although it would be otiose to deny that scientific ideas might more readily arise in some settings as against others) to realise that no theory can be entirely secure. In principle, new lines of evidence may lead to radical reformation or even the abandoning of a theory. But seldom, if ever, does one new observation have this consequence. In the case of Darwin one can be as sure as possible that while there is much about evolution yet to be discovered (and if Darwin was with us, he surely would have been delighted to acknowledge this), but that its foundations are secure.

Bronze sculpture of Charles Darwin as a young man by Anthony Smith. The sculpture, which was unveiled in 2009, is located in the grounds of Christ’s College, Cambridge (Image: Used with the kind permission of the ... moreMaster and Fellows of Christ’s College, Cambridge)
What of Darwin the individual? It is easy to sink into hagiography, although by most accounts he had many admirable characteristics. Nevertheless, one can note he oscillated between times of exceptional excitement and others of introspection, even pessimism. He was patient (up to a point) with critics, but acerbic with those who he judged (perhaps not always fairly) to have misunderstood his ideas. What surely marks him as almost entirely original is his extraordinary ability to ‘join the dots’, to see how apparently unrelated facts made sense from a wider perspective.
So too, Darwin knew that his theory was vulnerable, with weak points, but as has often been noted, when these were ultimately resolved the overall theory was strengthened. Stories of a return to his Christian faith on his death-bed may not be as misplaced as have generally been thought, but there is little doubt that he had little time for the apparent niceties of religion. As is often the case with great thinkers, Darwin had a grand vision that was perhaps more akin to a deist, even a pantheist, but significantly he never lost his sense of wonder, even awe. Was Darwin good at everything? No! When it came to philosophical skills he himself admitted that his abilities were limited, but was content to emphasize the empirical data that spoke to him more clearly than anything else. Nobody can do everything, and Darwin remains a giant upon whose shoulders we now see a little further.
Text copyright © 2015 Victoria Ling. All rights reserved.
References
Adams, D.N. (1988) The Long Dark Tea-Time of the Soul. Pan Books.
Desmond, A. and Moore, J.R. (1991) Darwin. Penguin.
Ronald A. Fisher

Photograph of Ronald Fisher taken in 1946 by Walter Stoneman. Stoneman took many photographs of Fellows on behalf of the Royal Society (Image: Used with the kind permission of the Royal Society of London)
Sir Ronald Aylmer Fisher (1890-1962) was an evolutionary biologist and statistician. He is perhaps best known for inventing an array of revolutionary statistical procedures that could be used within the natural sciences. These include:
– ANOVA (analysis of variance), which is a test that allows the identification of significant differences between three or more samples so long as the populations from which these derive follow the normal distribution and exhibit homogenous variances;
– Extreme Value theory, a statistical sub-discipline that assesses the probability of extreme events occurring;
– P-value, in effect the probability with which we accept a mistake when we reject a null hypothesis that is actually valid. In other words, a p-value equal to 0.05 (which is the standard) means that when we conclude that there is no statistically significant difference between two or more samples (that is, when we reject the null hypothesis) there is 5% chance that there is indeed a significant difference but we missed it.
Fisher’s statistical techniques revolutionised the way data could be queried and analysed, enabling scientists to test hypotheses in an entirely objective way and allowing us to explore patterns in massive datasets where any qualitative visual analysis is impossible. However, Fisher’s contribution to our understanding of evolution goes far beyond the statistical tests with which he is most widely and commonly associated.
The world-renowned statistician and geneticist Professor Anthony Edwards was the last undergraduate that Fisher accepted. When asked why Ronald Fisher deserved to remembered as the great scientist that he was, Edwards responded that it was a difficult question to answer because Fisher was:
“..one of those people whose work is at a level of subtlety that it’s really quite difficult to explain why he should be revered so much as he is – as a great scientist. It’s one thing to split an atom, or discover the electron, or do the other things for which Nobel Prize winners around Cambridge are known for. But it’s quite another thing to work at the level of subtlety that Fisher worked at – both in statistics and in evolutionary biology.” (pers. comm. 2014)
An even more challenging question we asked Edwards was what, in his opinion, were Fisher’s three most significant contributions to science. Edwards stated that if one is to identify three key contributions from Fisher’s work, then one has to think in terms of which publications you would wish to draw people’s attention to. Edwards’ suggestions are described here in his own words:
(i) “Statistical Methods for Research Workers from 1925, which ran to 40 editions and which completely changed the map of statistical practice in science from that time on. And that’s probably the best known of his contributions. Of course, it relies so much on work that he himself did in the preceding ten years or so, so at the time it was extraordinarily novel. There’s a paper from 1922 called On the mathematical foundations of theoretical statistics which really sets the scene for the writing of this book.”
(ii) “The Genetical Theory of Natural Selection from 1930. That is an extraordinary book because it’s really only now, and I do mean now – the last few years, that people have – in evolutionary biology – have actually got around to reading it carefully. Now of course it always had its followers, but they’ve been few and far between. So, there is now work coming out of the thoughts in that book, 84 years after it was written and so much of what happens in modern evolutionary biology can be traced either directly to that book, or to people whose names you’ll be familiar with, particularly W.D. Hamilton whose ideas come from that book anyway and have been made extended and made so well known by people of the next generation, but there’re few and far between. So, the number of serious readers of that book from 1930 to about 2005 you could count on the fingers of one hand. And indeed it was one of Charles Darwin’s sons, Leonard Darwin, who was a great supporter of Fisher, a great friend of Fisher’s, who warned Fisher that his great book would be extremely well known in the long-term but it really would take quite a long time, and that’s exactly what’s happened.”
(iii) “On the dominance ratio. Nobody had a finer understanding of what Darwin was saying than Fisher. Whether he could communicate that and extend it is a different question, but nobody had a finer understanding….So, what should it [the third point] be? Well I think we ought to go back to before 1930 and look at the year 1922. And in that year Fisher published a paper called “On the dominance ratio”. And it’s a most extraordinary paper, it’s really the foundation paper for population genetics and mathematical genetics because it does so many things: it proves for the first time the stability of a genetic system if the heterozygotes are at an advantage over the homozygotes. And that’s very fundamental to mathematical genetics. It introduces what later came to be known as the Wright-Fisher model – for modelling stochastic processes in genetics. Quite wrongly called the Wright-Fisher model because Fisher introduced it all by himself and it wasn’t until 1931 that Sewall Wright wrote about it.”
In many ways, Fisher’s contribution to science was not appreciated in his own time. The sheer depth of his knowledge and the subtle level at which he worked, meant that to a large extent he was academically isolated.
When asked why it has taken the biological world so much longer to appreciate Fisher’s work than the statistical world, Edwards remarked: “Well, it was partly Fisher’s fault, because he made this one huge contribution of writing “The Genetical Theory of Natural Selection” and he wrote it in his beautiful English, with a lot of mathematics hidden behind the English, because he thought that he wanted to express his views to biologists who were not at home with the mathematics. And a consequence of this is that the mathematicians ignored it and the biologists didn’t understand it. But nowadays we have a much greater number of people in biology who can do the mathematics and there have been many studies over the years recreating the mathematics which lies behind Fisher’s verbal descriptions in The Genetical Theory.”
FortyTwo extends its appreciation and thanks to Professor Anthony Edwards.
Text copyright © 2015 Victoria Ling. All rights reserved.
Barbara McClintock
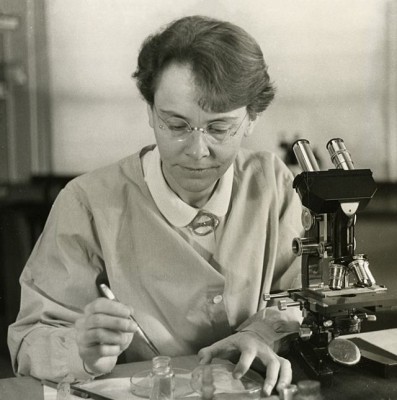
Barbara McClintock in her laboratory in 1947 (Image from the Smithsonian Institution collection via Wikimedia Commons)
Barbara McClintock (1902-1992) was an American geneticist and a pioneer in the field of cytogenetics, a branch of genetics that focuses on the function of chromosomes in individual cells. McClintock’s work on chromosomes in maize revolutionised this field. In her time, she was widely respected, receiving a number of prestigious awards and fellowships, not least the National Medal of Science. However, it is her discovery of transposons – which revolutionised our understanding of genetics – that was entirely radical. Yet it took thirty years for the scale of her discovery to be fully acknowledged, with the award of the Nobel Prize in Medicine or Physiology “for her discovery of mobile genetic elements” in 1983 (NobelPrize.org).
A trademark of McClintock’s research was her unwavering attention to detail. Meticulous data analysis remains essential if one is to have confidence in one’s scientific hypotheses, and all the more vital if such assertions are novel and so question established ideas. Challenging the status quo is precisely what McClintock’s discovery of transposons achieved.
It was her thorough interrogation of the data that enabled her to have first confidence and eventually conviction as to what at that time seemed to be an unlikely – even implausible – scenario. In effect, her data showed that genes could be mobile, moving around the chromosomes, hopping from one place and re-inserting themselves elsewhere. McClintock discovered these peculiarly behaving genes whilst working on maize plants in the late 1940s. We now call these genes ‘transposons’, often aptly referred to as ‘jumping genes’. In November 1953 McClintock published her findings in the journal Genetics, with a paper entitled Induction of instability at selected loci in maize.
McClintock’s discovery did not sit comfortably with the twentieth century consensus as to how genes should behave. At the time, geneticists considered that genes were stable entities and the notion that there could be ‘renegade’ strands of DNA moving about the genome was treated with extreme caution. But if one places McClintock’s discovery in a broader social and historical perspective, given the nature of the proposal – that is, the idea that something which was perceived as stable, was actually subject to change – one could almost see the skepticism with which it was initially received as less surprising, maybe even predictable.
In some broad sense this might say something about a human desire for stability. If one looks back over the history of science it is not unreasonable to suggest that the scientific theories which met with the most vehement, almost visceral resistance were often those which are not only ‘big ideas’, but those which have championed ‘change’ in a previously accepted framework of ‘permanency’.
This is all the more true when it comes to natural phenomena that an individual cannot observe with the naked eye and/or witness over the course of their own short life-span. Notable examples include the idea of the Big Bang, and nearer to home that of continental drift; a proposal by the meteorologist Alfred Wegener who suggested (quite rightly) that Earth’s continents were once joined together and slowly drifted apart (and continue to move) over millions of years. From the time it was first proposed in 1912 the idea of continental drift found little favour and until the late 1960s the consensus remained that the Earth’s continents were static.
Another example is of course Charles Darwin’s theory of evolution by natural selection, which was proffered at a time when society at large was still committed to the notion of the permanency of species (i.e. that each species on Earth was created in its current form and was not the result of evolution). Here too, Darwin was correct, but some of the resistance stemmed from people who saw weaknesses in the theory, and ones that Darwin lost little time in addressing.

Pigmentation on corn kernels reveals the activity transposons (Image: Damon Lisch via Wikimedia Commons)
In the case of McClintock her work perhaps reflects more than a hint of our collective human condition inasmuch as although the existence and behaviour of transposons was relatively swiftly accepted by other geneticists who conducted research on maize, when it came to applying these ideas to other forms of life, not least humans, the implications were far more slowly accepted. In the case of transposons, interest in these small pieces of mobile DNA remained largely dormant until the 1970s when they were found in viruses and bacteria. From there, interest was ignited and further research revealed that transposons are found in most life-forms, including of course humans.
Let us leave the last words to McClintock. After receiving the Nobel Prize in 1983 she remarked: “You just know sooner or later, it will come out in the wash, but you may have to wait some time.” (quoted in McGrayne 2001, p.144)
Text copyright © 2015 Victoria Ling. All rights reserved.
References
McClintock, B. (1953) Induction of instability at selected loci in maize. Genetics 38, 579–99.
McGrayne, S.B. (2001) Nobel Prize Women in Science. Carol Publishing Group, Secaucus, NJ.
Pray, L. and Zhaurova, K. (2008) Barbara McClintock and the discovery of jumping genes (Transposons). Nature Education 1, 169.
Ravindran, S. (2012) Barbara McClintock and the discovery of jumping genes. Proceedings of the National Academy of Sciences, USA 109, 20198-20199.
Gregor Mendel
Johann Mendel (1822-1884) was an Austrian botanist, now widely regarded as the father of modern genetics. He was given the name ‘Gregor’ – by which he is more commonly known – when he joined the Augustinian monks. It is worth remembering that the idea that all religious people are de facto opposed to science, let alone evolution, is simply incorrect, and Mendel is a reminder that a keen intellect and life in a monastery (the Abbey of St. Thomas) are not exclusive possibilities.
Mendel is most famous for his discovery of the basic principles of genetic heredity through experiments with pea plants (Pisum sativum). Pea plants were good subjects for study because their physical characteristics are relatively few and simple, and fertilisation is easily controlled. Today, Mendel’s findings are ranked amongst the greatest in biology, and our basic understanding of how traits are inherited from one generation to the next comes from the principles he proposed.
In 1865 Mendel presented what would later come to be regarded as a seminal paper in the history of science, Versuche über Pflanzenhybriden (Experiments on Plant Hybridization) to the Natural History Society of Brno in Moravia, and it was published the following year. In it, Mendel laid down three main principles of inheritance:
– Principle of segregation: in diploid species each individual possesses two types of (allele) gene for each trait. A parent will only pass one of these versions onto offspring. The one that gets passed on is in principle random.
– Principle of independent assortment: during gamete formation, each combination of alleles stands an equal chance of occurring.
– Fundamental theory of heredity: discrete units of inheritance are passed from parents to offspring. Today, we call these ‘units of inheritance’ genes.
In the nineteenth century, most biologists believed that offspring inherited a ‘blended’ set of traits from both parents. So, for example, the offspring of a tall man and a short woman would be expected to produce a child of medium height. This didn’t, however, quite add up and notably Charles Darwin had struggled to account for the mechanism by which traits were passed from one generation to the next. Indeed, a coherent theory of heredity was notably absent from On The Origin of Species.
In his 1868 work The Variation of Animals and Plants Under Domestication, Darwin proposed a theory called ‘pangenesis’ which proposed that every cell in the body combined to influence the constitution of offspring. As we now appreciate, this idea was deeply flawed and would eventually be replaced by Mendel’s theory of inheritance, but it highlights just how difficult it was to account for the inheritance of traits in a pre-gene informed world, and as such, how insightful Mendel’s principles proved to be.
In stark contrast to Darwin’s pangenesis theory, Mendel showed that when one variety of pure-bred plant was cross-pollinated with another, the offspring resembled either one or the other of the parent plants; traits were passed from parent to offspring intact, not as a blend of the two. Mendel further demonstrated that some traits are dominant and some are recessive. Dominant traits essentially mask the effect of a recessive trait. By doing so, Mendel highlighted that the subject of inheritance was clearly at one and the same time more complicated and also more simple than had hitherto been suspected by theories propounding ‘blending’. Whilst it is now clear that the processes of genetics are almost infinitely more complex and sophisticated than Mendel could ever have realized, his discovery was the lynch-pin for the emergence of neo-Darwinism.
As pointed out by Henig (2000), even though a reprint of Mendel’s 1866 paper was found in Darwin’s library, it appears that Darwin was unaware of Mendel’s work and may not have even read it. Darwin may not have been impressed. He was already familiar with the work of the French botanist Charles Naudin, who had reached many of the same conclusions as Mendel but without the statistical support.
But it wasn’t just Darwin who failed to pick up on the significance of Mendel’s research; for nearly forty years his 1866 paper remained largely unacknowledged. It was not until after Mendel’s death that his work was re-discovered by the scientific community. In 1900, the botanists Hugo de Vries, Carl Correns and Erich von Tschermak independently published work within a two month span of each other that acknowledged Mendel’s research. Biologists then became interested in the work of this virtually unknown man, and the wider the paper became known, the more scientists of the day wanted to learn about him.
But it has not all been plain sailing; with re-discovery there has also been controversy. Most notably, there has been debate in recent years about whether or not Mendel’s results were artificially modified (not necessarily by Mendel himself, but possibly by an assistant) in order to fit with his expectations, a suggestion which largely stems from Ronald Fisher’s (1936, p.132) assertion that:
“..the data of most, if not all, of the experiments have been falsified so as to agree closely with Mendel’s expectations.”
As is neatly explained by Novitski (2004), most scientists agree that the results from Mendel’s garden pea experiments conform more closely with theoretical expectations (with ratios such as 3:1, 1:2:1 etc.) than one based upon chance, thus arguably making them too good to be true. Since then, many people have studied and replicated Mendel’s garden pea experiments, analysed the outcomes, and debated the ratios. On moral grounds, there is no doubt that Fisher took the ethically and academically correct route by voicing his concerns, but the emerging consensus is that the criticism is unfounded. To quote Fairbanks and Rytting (2001, p.751):
“There is no credible evidence to indicate that Mendel was inaccurate or dishonest in his description of his experiments or his presentation of data. The main questions about his results can be resolved by an appeal to botanical principles and historical evidence.”
As succinctly stated by Tudge (2000, p.286) “..the complexities of modern genetics, and all the ramifications, flow naturally from Mendel’s initial notions..”. In addition, Mendel must be given particular credit for the fact that he formed his hypotheses in an era long before genes had been identified as the unit of inheritance or the structure of DNA had been discovered. He drew his conclusions from observing the evidence in front of him, and that weight of evidence led him to break with orthodoxy and challenge (albeit unacknowledged at first) commonly held perceptions regarding the inheritance of traits. Mendel laid the groundwork that made it possible for us to understand the basic form of genetic inheritance, and in doing so he founded the field of genetics which today has huge benefits for humankind; not least in terms of understanding diseases and developing medicine.
Today we would like to think that new ideas will quickly surface, and certainly given the internet and social networks all this is greatly facilitated. Even so, ideas are ideas and without the fertile soil of an open, curiosity-driven intellect they will wither and die.
Text copyright © 2015 Victoria Ling. All rights reserved.
References
Darwin, C. 1868. The Variation of Animals and Plants Under Domestication. London: John Murray.
Fairbanks, D.J. and Rytting, B. 2001. Mendellian controversies: A botanical and historical review. American Journal of Botany 88 (5), 737-752.
Fisher, R.A. 1936.Has Mendel's work been rediscovered? Annals of Science 1, 115–137.
Hartl, D.L. and Fairbanks, D.J. 2007. Mud sticks: on the alleged falsification of Mendel’s data. Genetics 175, 975–979.
Henig, R.M. 2000. The Monk in the Garden: The Lost and Found Genius of Gregor Mendel, the Father of Genetics. Boston: Houghton Mifflin.
Mendel, J.G. 1866. Versuche über Pflanzenhybriden Verhandlungen des naturforschenden Vereines in Brünn, Bd. IV für das Jahr, 1865. Abhandlungen, 3–47.
Novitski, E. 2004. On Fisher’s Criticism of Mendel’s Results With the Garden Pea. Genetics 166, 1133-1136.
Sandler, I. 2000. Development: Mendel’s Legacy to Genetics. Genetics 154, 7-11.
Tudge, C. 2000. In Mendel’s Footnotes: An introduction to the science and technologies of genes and genetics from the nineteenth century to the twenty second. London: Jonathan Cape.
Vítĕzslav, O. 1996. Gregor Mendel: The first geneticist. Oxford, New York, Tokyo: Oxford University Press. (Translated by Stephen Finn)
George Gaylord Simpson

George Gaylord Simpson in 1965 (Image from Concession to the Improbable: An Unconventional Autobiography via Wikimedia Commons)
George Gaylord Simpson (1902-1984) was an American palaeontologist. He published extensively on the taxonomy of extinct and extant animal species, the intercontinental migration of animals, and helped to reconcile the fields of palaeontology and genetics.
One of Simpson’s greatest contributions to evolutionary studies was as one of the founding fathers of the ‘modern synthesis’. In the early twentieth century the study of evolution experienced a renaissance with the work of Gregor Mendel. Even so, some considered that Mendel’s law of genetic inheritance was irreconcilable with the Darwinian theory of evolution. That is, evolution by natural selection asserted that new traits can appear in a species that were not previously present. This conflicted with Mendelian genetics which stated that genes – although passed on to offspring in different combinations – remained unchanged through the generations.
However, in the early 20th century, an increasing amount of research was carried out on how genes ‘mutate’. Mutation creates permanent changes to genes and can lead to new traits in a given species which have never previously been seen; totally harmonious with evolution by natural selection. The modern synthesis argued that Darwin’s theory and Mendel’s Law were complimentary pieces of the same jigsaw puzzle. It stressed that whilst Darwinian evolution identified the importance of natural selection in evolution, changes in the gene pool are also caused by genetic drift, mutation and gene flow.
Simpson was especially keen to integrate the fields of palaeontology and genetics, and this was elegantly demonstrated in his 1944 publication Tempo and Mode in Evolution. He used palaeontology to show that evolution was not ‘goal-oriented’ (as was being argued at the time by those who thought Darwinian theory could not be reconciled with Mendelian inheritance). In particular, he used the evolutionary history of the horse to show that evolution is not a straightforward, linear process, but one that is littered with extinctions. Broadly speaking, the modern synthesis shows how different academic disciplines – in this case, initially palaeontology and genetics – come together to answer scientific questions common to them both. As the decades have passed, academia in general and evolutionary studies in particular, have become increasingly multi-disciplinary; it takes information from a broad range of disciplines to build a thorough understanding of the evolutionary process.
Simpson can also be noted for his forceful opposition to Alfred Wegener’s theory of Continental Drift. Continental drift – which, in the form of plate tectonics, is now an accepted fact –is the idea that the Earth’s continents have moved over millions of years. This in turn explains why we find very similar fossils on continents that are today separated by vast oceans. Simpson however, argued that the continents were fixed, arguing that the distribution of extinct creatures could be explained by processes other than moving landmasses. Such was the respect for Simpson, together with the influence of his publications that this led to the theory of Continental Drift losing credibility in the scientific world.
However, by the 1960’s geophysical evidence began to support – and ultimately validated – Wegener’s theory of Continental Drift, and this in turn prompted Simpson to revise his stance. In doing so, Simpson demonstrated what the scientific method is all about; testing a hypothesis, holding it up to scrutiny and, if need be, revising one’s own theory in the face of overwhelming facts. As a point of interest, it should be noted that Simpson’s idea of continental immobility, although wrong in the broad sense, had several fruitful aspects, such as the idea of “island hopping”, “rafting” and that Antarctica once served as a land corridor from South America to Australia. So too our understanding of the evolution of South America’s biotas very largely hinges on first its isolation, with occasional episodes of rafting from Africa, before connectivity was made via the Isthmus of Panama, first as island hopping and then a corridor. Animals moved in either direction and that explains why we see opposums in Toronto and llamas in Chile.
Text copyright © 2015 Victoria Ling. All rights reserved.
References
Eldredge, N. (1985) Unfinished Synthesis: Biological Hierarchies and Modern Evolutionary Thought. Oxford University Press.
Frankel, H. (1987) The continental drift debate. In Scientific Controversies: Case Studies in the Resolution and Closure of Disputes in Science and Technology (H.T. Engelhardt Jr and A.L. Caplan, eds), pp. 203-248. Cambridge University Press.
Laporte, L.O.F. (1994) Simpson on species. Journal of the History of Biology 27, 141–159.
Olson, E.C. (1991) George Gaylord Simpson. 1902-1984. A biographical memoir by Everett Olson. Biographical Memoirs of the National Academy of Sciences 60, 331–353.
Simpson, G.G. (1943) Mammals and the nature of continents. American Journal of Science 241, 1-31.
Simpson, G.G. (1944) Tempo and Mode in Evolution. Columbia University Press.
Nikolai Vavilov
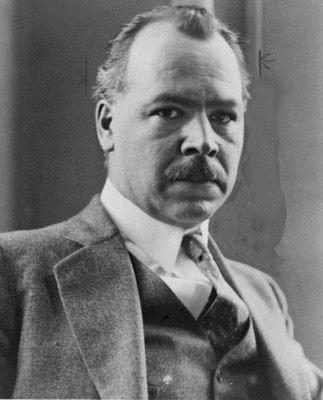
Nikolai Ivanovich Vavilov (Image: Library of Congress reproduction number LC-USZ62-118109/Wikimedia Commons)
Nikolai Ivanovich Vavilov (1887-1943) was a Russian botanist, geneticist, and agronomist. He was President of the National Geographic Society of the USSR, set up the Department of Genetics at the All-Union Institute of Plant Breeding in what was then Leningrad, and founded what was once the world’s largest collection of plant seeds, collected from every corner of the world.
Vavilov had a contagious enthusiasm for science and his contribution to evolutionary studies was immense, yet he has largely passed under the radar in terms of the wider public and scientific appreciation. Not only have his scientific contributions failed to be given the wider acknowledgement they so readily deserve, but his premature death was shameful; in 1941 he was imprisoned for criticising anti-Mendelian theories that were supported by the Stalin regime, and he died of starvation in the Saratov prison two years later.
Vavilov’s main research interest was in cultivating crops such as wheat and corn in order to tackle famine in Russia and other parts of the world. Specifically, he wanted to use the new science of genetics to breed varieties of crop that not only yielded more grain, but that would also withstand extreme temperatures and be more resistant to insects. As described by Ilya Zakharov (2005), in order for Vavilov to accomplish this goal he needed to overcome two interrelated hurdles. The first was the identification and collection of plant samples from across the globe in order to study and harness their genetic potential. The second was the conservation of the diverse range of wild and domesticated plants in their native setting, the diversity of which Vavilov believed was being eroded as a result of the destruction of natural habitats. Presciently, he spoke about the ‘geography of genes’ and the study of the distribution of genes is now a flourishing area of scientific research, both in plants and animals (including humans).
One of his seminal papers, published in 1912, was in Genetics and Agronomy, where he argued that Mendelian genetics could be used as a basis for the cultivation of plants. Throughout the 1920s and 1930s, Vavilov made expeditions to 40 countries, spanning five continents in order to collect samples from cultivated plants. This was an immense undertaking, particularly given that many of the countries he visited were politically unstable, making such excursions decidedly risky.
Indeed, for his expedition to Afghanistan in 1924 the Russian Geographic Society awarded him a medal in recognition of his ‘Exploits in Geography’. At the time, his work was widely acknowledged, not only in Russia but across Europe, and just before the First World War he worked in leading laboratories in Britain, France and Germany. Vavilov established the world’s first international seed bank of food plants, containing hundreds of thousands of specimens and preserved within that was the genetic diversity which could be used to breed the high-yield, high-resilience crops which were a test of his hypotheses.
Vavilov was ahead of his time in recognizing the importance of preserving genetic diversity. For example, he identified seven primary centres of origin for the world’s main crops. Centres of origin are geographical areas that have been identified as the original source of specific crop plants. It is from these primary sources that crops were domesticated. The identification and preservation of these regions is essential for the cultivation of crops today because not only are they important sources for the plants’ genes, but highlight areas where biosafety measures should be considered when it comes to introducing genetically modified crops and/or habitats threatened with destruction.
Scientifically, it can be argued that Vavilov’s work on crops and genetic diversity was not only linked to, but also overshadowed, by his profoundly interesting idea of ‘Law of Homologous Series’, first published in the Journal of Genetics in 1922. The starting point was to emphasise the importance of the initial genotype and its subsequent variability. As highlighted by Peter Pringle (2009, p.71): “Darwin was the first to note that similar, sometimes even identical, characteristics arise in animals and plants. On the banks of the River Plate between Uruguay and Argentina, Darwin saw bulldog-faced cows that, because of their jaw, resembled certain breeds of dogs and pigs. But Darwin could take his observation no further because genetics, the science of heredity and variation, did not exist”. Vavilov’s Law of Homologous Series, however, essentially picks up where Darwin left off. To further quote Pringle (2009, p.71):
“Vavilov laid out a simple rule for hunters of crop plants: similar features, such as stem size, and leaf size and shape, could be found in the various evolutionary stages of all closely related species, genera, and even families.”
In 1940 Vavilov was arrested by the ruthless Stalin regime for his criticism of the anti-Mendelian concepts of the Soviet biologist Trofim Lysenko, which happened to be favoured by Stalin. This meant that scientific dissent from Lysenko’s theories, regardless of how absurd Lysenko’s claims may have been, was met with persecution and even a death sentence. Vavilov was sentenced to death in 1941, but in 1942 this was amended to twenty years imprisonment. In 1943 he died in prison of starvation. So great was Lysenko’s political influence that in 1948 the Lenin Academy approved ‘Lysenkoism’ as the accepted direction of biology for the Soviet Union.
Following the death of Stalin in 1953 there was a review of death sentences carried out under his authority, and in 1955 the verdict against Vavilov was rescinded by the Military Collegium of the Supreme Court of the Soviet Union. Despite increasing criticism from scientists across the globe, Lysenko managed to maintain his powerful position, and it was not until 1965 that he was forced to resign as Director of the Institute of Genetics. Following that, in 1967, the All-Union Institute of Plant Industry was re-named the ‘N.I. Vavilov Institute of Plant Industry’. By this time, Vavilov was considered to be one of the great names in Soviet science. Far too late, but at least some recognition of a great biologist. In the words of Zakharov (2005, p.301):
“The whole life of Nikolai Ivanovich Vavilov is a remarkable example of wholehearted devotion to science, to his homeland and to humanity.”
Text copyright © 2015 Victoria Ling. All rights reserved.
References
Crow, J.F. (1993) N.I. Vavilov, Martyr to Genetic Truth. Genetics 134, 1-4.
Crow, J.F. (2001) Plant breeding giants: Burbank, the Artist; Vavilov, the Scientist. Genetics 158,1391–1395.
Pringle, P. (2009) The Murder of Nikolai Vavilov. JR Books.
Tzotzos, G.T., Head, G.P. and Hull, R. (2009) Genetically Modified Plants: Assessing Safety and Managing Risk. Elsevier.
Vavilov, N. I. (1922) The law of homologous series in variation. Journal of Genetics 12, 47-89.
Zakharov, I.A. (2005) Nikolai I Vavilov (1887–1943). Journal of Biosciences 30, 299–301.